The Interstate Technology & Regulatory Council (ITRC) has coined the term Natural Source Zone Depletion (NSZD) to describe the natural loss of Light Non-Aqueous Phase Liquid (LNAPL) due to various processes, the most important of which is biodegradation (ITRC, 2009).
A series of research projects have determined that this depletion is occurring at much faster rates than first thought, up to 100’s to 1000’s of gallons per acre per year (Johnson et al., 2006; Lundegard and Johnson, 2006; Sihota et al., 2011; McCoy et al., 2015). Additionally, Garg et al. (2017) describes an overview of the latest research and key processes controlling NSZD.
What is Thermal NSZD®?
Thermal NSZD® is a continuous thermal monitoring system that is used to estimate the daily and cumulative mass of LNAPL destruction in the subsurface by microbial activity (Horst et al., 2017; Sale et al., 2018). The conversion of net temperature change to mass degradation is based upon a series of thermodynamic equations developed by Colorado State University (US Patent No. 10,094,719). Thermal NSZD® measures the heat generated by biodegradation processes using a vertical series of thermocouples, which can then be converted to a biodegradation rate. Temperatures are corrected from a non-impacted background location to remove seasonal temperature effects in the subsurface. The following illustration summarizes the conceptual model for NSZD at hydrocarbon sites based on recent studies and the current understanding of biodegradation:
Example heat signal cross-section from a site (Source: Colorado State University, Stockwell, 2015):
The red area in the figure shows the net heat (additional heat compared to background location) generated by LNAPL degradation from four stations, each with up to seven thermocouples distributed vertically to 40 feet below ground surface.
Several other studies have discussed the heat signal resulting from biodegradation, with the following key findings:
- The temperature in an LNAPL-impacted location is consistently higher than a background well, typically ranging from 2 to 2.5ºC. At locations near an active Soil Vapor Extraction (SVE) system, temperatures are even higher than LNAPL-impacted locations. This increase in temperature is consistent with enhanced biodegradation due to influx of air through the subsurface (Sweeney and Ririe, 2014).
- Temperature measurement of subsurface heat production in LNAPL zones is attractive because high-resolution, continuous data can be obtained at a low cost; it is also applicable at sites with surface covers that may prevent adequate soil gas flux (Suthersan, 2015).
- Temperatures in the unsaturated zone above an LNAPL body were up to 2.7ºC above background locations. Additionally, the annual average temperature for each location was used to determine the amount of heating from methane oxidation. Using Fourier’s First Law, degradation rates were estimated from each location using two different methods. Overall, the rates estimated from temperature measurements were comparable to rates determined from an independent study using surface CO2 efflux data (Warren and Bekins, 2015).
- Biodegradation rates have been known to vary with factors such as temperature (Sihota et al., 2016; Kulkarni et al., 2017), availability of nutrients, as well as many others (Garg et al., 2017). As such, continuous measurements from Thermal NSZD® can provide a more accurate estimate of long-term NSZD rates (Horst et al., 2017).
How to Apply Thermal NSZD® at Your Site
Application of the Thermal NSZD® technology involves the installation of Thermal Monitoring Stations and set-up of the stations on the Thermal NSZD® Dashboard.
Install Thermal Monitoring Stations
Install 5-10 Thermal Monitoring Stations per site in an LNAPL zone and at least one unimpacted background location. Each station consists of:
- Vertical profile of thermocouples that are installed by Direct Push or conventional drilling rigs
- Datalogger and wireless communication system powered by solar panel
- Weather-proof enclosure for monitoring electronics

Thermocouple
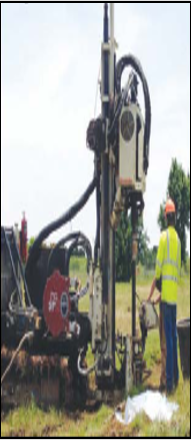
Installation via Direct Push Rig
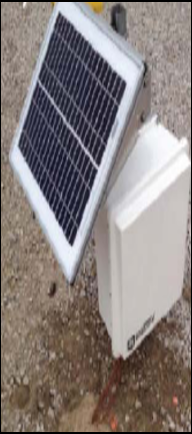
Datalogger and wireless communication system
Thermal NSZD® Dashboard
Methodology to obtain NSZD rates:
- Wireless system automatically transmits subsurface temperature data from each monitoring station to the Thermal NSZD® Dashboard
- Temperatures are corrected from background values to obtain net temperatures (background-corrected temperatures) per LNAPL-impacted location
- NSZD rates are calculated using heat conduction principles to obtain a heat flux (qh) to ground surface
(Points 1 and 2) and saturated zone (Points 3 and 4). Specifically, heat flux is calculated as follows:qh = ‐KT (ΔT/Δz)
where qh is the heat energy lost across a surface (W/m2), KT is the thermal conductivity (W/mºC), ΔT is the change in
temperature (ºC), and Δz (m) is the depth interval across which heat flux is calculated. The total heat flux is converted to an NSZD rate (in gallons LNAPL degraded per acre per year) using the thermodynamics of the reactions involved in biodegradation.
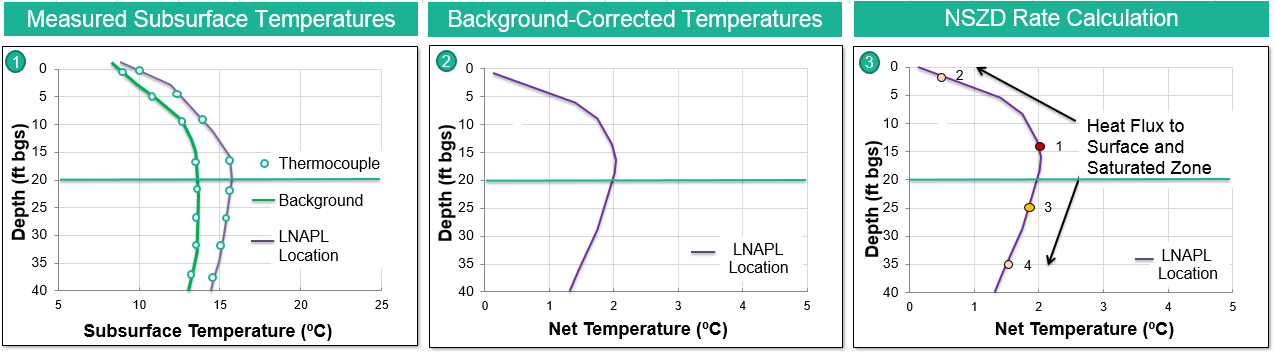
Dashboard interface provides:
- Temperature vs. time and temperature vs. depth for each station
- Daily NSZD rates (gallons/acre/year) per station
- Site-wide cumulative NSZD rates updated daily
Key Benefits of Thermal NSZD®
- One-time installation of Thermal Monitoring Stations, with minimal operations and maintenance (O&M) and no additional site visits required for sampling events
- Quantitative measure of on-going NSZD rates, which can be used to compare against performance of other active remediation technologies
- Continuous, remote monitoring of NSZD rates
- Off-the-shelf, commercially-available components
- Lower costs when compared to conventional monitoring methods and other LNAPL remediation technologies
Garg, S., Newell, C.J., Kulkarni, P.R., King, D.C., Adamson, D.T., Renno, M.I., and Sale, T., 2017. Overview of Natural Source Zone Depletion: Processes, Controlling Factors, and Composition Change. Groundwater Monitoring & Remediation, 32(3): 62-81. doi: 10.1111/gwmr.12219. Available at: http://onlinelibrary.wiley.com/doi/10.1111/gwmr.12219/full
Horst, J.F., Welty, N., Schnobrich, M., Sinha, P., Kulkarni, P., 2017. Digital Innovation: The Next Disruptive but Transformative Remediation Frontier. Groundwater Monitoring & Remediation, 37(3): 19-27. doi: 10.1111/gwmr.12222. Available at: http://onlinelibrary.wiley.com/doi/10.1111/gwmr.12222/abstract
ITRC (Interstate Technology Regulatory Council), 2009. Evaluating Natural Source Zone Depletion at Sites with LNAPL . Washington, D.C.: Interstate Technology Regulatory Council, LNAPLs Team. Available at: http://www.itrcweb.org/GuidanceDocuments/LNAPL-1.pdf
Johnson, P., Lundegard, P. and Liu, Z., 2006. Source Zone Natural Attenuation at Petroleum Hydrocarbon Spill Sites—I: Site-Specific Assessment Approach. Groundwater Monitoring & Remediation, 26:82–92. doi:10.1111/j.1745-6592.2006.00114.x. Available at: http://onlinelibrary.wiley.com/doi/10.1111/j.1745-6592.2006.00114.x/abstract
Kulkarni, P.R., King, D.C., McHugh, T.E., Adamson, D.T., Newell, C.J., 2017. Impact of Temperature on Groundwater Source Attenuation Rates at Hydrocarbon Sites. Groundwater Monitoring & Remediation, 37(3): 82-93. doi: 10.1111/gwmr.12226. Available at: http://onlinelibrary.wiley.com/doi/10.1111/gwmr.12226/abstract
Lundegard, P. D. and Johnson, P. C., 2006. Source Zone Natural Attenuation at Petroleum Hydrocarbon Spill Sites—II: Application to a Former Oil Field. Groundwater Monitoring & Remediation, 26:93–106. doi:10.1111/j.1745-6592.2006.00115.x. Available at: http://onlinelibrary.wiley.com/doi/10.1111/j.1745-6592.2006.00115.x/abstract
McCoy, K., Zimbron, J., Sale, T. and Lyverse, M., 2015. Measurement of Natural Losses of LNAPL Using CO2 Traps. Groundwater, 53: 658–667. doi:10.1111/gwat.12240. Available at: http://onlinelibrary.wiley.com/doi/10.1111/gwat.12240/abstract
Sale, T. C., Stockwell, E. B., Newell, C. J., Kulkarni, P. R., 2018. Devices and Methods for Measuring Thermal Flux and Estimating Rate of Change of Reactive Material within a Subsurface Formation. U.S. Patent No. 10,094,719 filed 18 February 2015 . Issued 9 October 2018.
Sihota, N.J., Singurindy, O., Mayer, K.U., 2011. CO2-Efflux Measurements for Evaluating Source Zone Natural Attenuation Rates in a Petroleum Hydrocarbon Contaminated Aquifer. Environ. Sci. Technol., 2011, 45 (2), pp 482–488. doi: 10.1021/es1032585. Available at: http://pubs.acs.org/doi/abs/10.1021/es1032585
Sihota, N.J., Trost, J.J., Bekins, B.A., Berg, A., Delin, G.N., Mason, B., Warren, E., and Mayer, K.U., 2016. Seasonal Variability in Vadose Zone Biodegradation at a Crude Oil Pipeline Rupture Site. Vadose Zone Journal, 15 (5). doi: 10.2136/zj2015.09.0125. Available at: http://pubs.acs.org/doi/abs/10.1021/es1032585
Stockwell, E., 2015. Continuous NAPL Loss Rates Using Subsurface Temperatures. Thesis. Department of Civil and Environmental Engineering. Colorado State University.
Suthersan, S., Koons, B. and Schnobrich, M., 2015. Contemporary Management of Sites with Petroleum LNAPL Presence. Groundwater Monitoring & Remediation, 35:23–29. doi:10.1111/gwmr.12099. Available at: http://onlinelibrary.wiley.com/doi/10.1111/gwmr.12099/abstract
Sweeney, R. E. and Ririe, G. T., 2014. Temperature as a Tool to Evaluate Aerobic Biodegradation in Hydrocarbon Contaminated Soil. Groundwater Monitoring & Remediation, 34:41–50. doi:10.1111/gwmr.12064. Available at: http://onlinelibrary.wiley.com/doi/10.1111/gwmr.12064/abstract
Warren, E., Bekins, B.A., 2015. Relating Subsurface Temperature Changes to Microbial Activity at a Crude Oil-Contaminated Site. Journal of Contaminant Hydrology, 182: 183-193. doi: 10.1016/j.jconhyd.2015.09.007.Available at: http://www.sciencedirect.com/science/article/pii/S0169772215300292